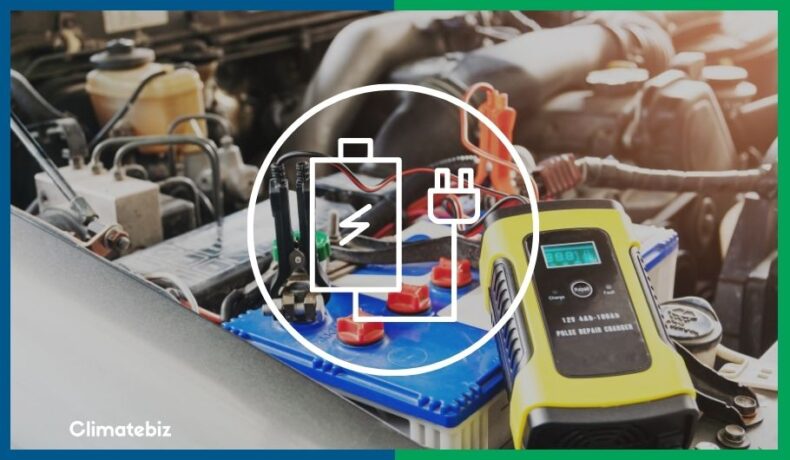
“What battery charger size is best for my needs?“
If you’re in the market for a new battery charger, you’ll quickly realize there are quite a few options to choose from. And with so many different chargers on the market, it can be hard to know which one is right for you.
When choosing a battery charger, the first thing you should consider is the battery charger’s size.
Battery chargers are rated in Amps (A), so by “battery charger size,” we’re referring to the charger’s maximum current output. And of course, the charger’s voltage should also be compatible with the battery’s voltage.
There are three main types of solar batteries: AGM, Gel, and LiFePO4. Each type has a specific charging profile; that is, they have different needs when it comes to charging specs.
Therefore, before choosing the right battery charger size, you need to consider the right charging specs for your type of battery.
In this article, we discuss how the charging profiles of these types of solar batteries differ from one another.
With this, you’ll understand why a specific battery charger size is more appropriate for one type of battery and not the other.
In addition, we present “battery charger size” charts so you know what size is best according to your battery’s type and capacity.
Finally, we answer the question, “Can a Battery Charger Be Too Big?”.
Affiliate Disclaimer
Table of Contents
What Battery Charger Size Do I Need?
As previously mentioned, battery chargers are rated in Amps (A). Therefore, “battery charger size” refers to the charger’s maximum current output.
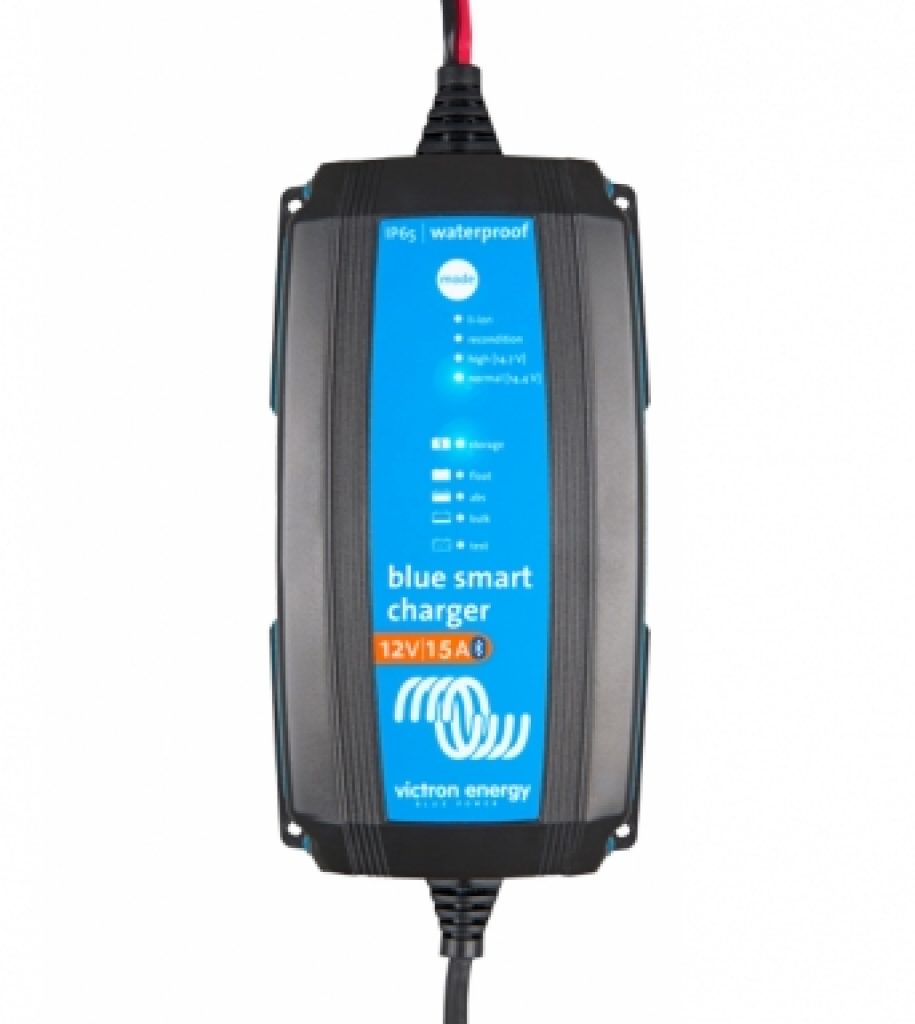
The correct battery charger for your needs is a charger that provides the optimal charging specs (charging voltage and current) for your battery.
By providing the optimal charging specs, your charger can:
- Efficiently charge the battery;
- Prolong the battery’s cycle life;
- Avoid overvoltage, overcurrent, and overcharge;
- Minimize charging time;
- Improve battery performance.
Will an improper charger charge your battery? Probably. However, you’ll notice a significant decrease in performance and service life over time.
“But what is the right battery charger for my battery?”
Well, it depends on your battery’s chemistry and total capacity. But before we jump into a proper explanation, you must understand what C-rate is.
What Is C-rate?
C-rate is the amount of charge/discharge current relative to batteries rated capacity. For example, if a battery is rated at 120Ah, 1C means 120A. Similarly, 0.5C means 60A, 0.2C means 24A, 0.1C means 12A and so on.
Charging C-Rate = Charging Current (A) / Battery Capacity (Ah)
Battery Chemistry Influences Charging Specs
To understand the proper way to charge your battery, you’ll need to learn about its chemistry.
Why? Because the charging profile — that is, how the charging specs vary throughout the charging process — is closely related to the battery’s chemistry.
With that in mind, let’s look at the charging profiles of the main types of solar batteries: Sealed Lead-Acid (SLA) batteries and LiFePO4 batteries.
Rechargeable Batteries
Batteries are an assembly of several electrochemical cells. The number of cells in a battery determines the battery’s voltage.
In rechargeable batteries, like solar batteries (LiFePO4 and SLA batteries), the chemical reactions within the electrochemical cells are reversible.
In general, a lead-acid cell has a nominal voltage of 2V. Therefore, a lead-acid battery with six cells has a nominal voltage of 12V (6 cells x 2V per cell = 12V).
LiFePO4 cells have a nominal voltage of 3.2V. Therefore, to achieve a 12V battery, four cells are connected in series, resulting in a nominal voltage of 12.8V.
Battery Cell’s Components
Each cell consists of two electrodes, a separator, and an electrolyte.
In lead-acid cells, the electrodes are Pb and PbO2, and the electrolyte is an aqueous solution of sulfuric acid. The separator’s material is different for each type of SLA (AGM and Gel).
In LiFePO4 cells, the electrodes are lithium iron phosphate (LiFePO4) and graphite, the separator is a polymer membrane, and the electrolyte is a solution of lithium salts dissolved in an organic solvent.
Discharging
During discharge, reduction and oxidation (or “redox”) reactions occur within each cell, converting chemical energy into electrical energy. This electrical energy powers the load connected to the battery.
Charging
During charge, an external source of power (like solar or a battery charger) is applied to push the redox reactions in the opposite direction (the least favorable direction).
Charging Voltage
The charging voltage needs to be a bit higher than the nominal voltage of the cell to kickstart the opposite redox reactions,
For example, in lead acid batteries, the nominal voltage of each cell is 2V. Therefore, the minimum charging voltage needs to be around 2.25V to 2.3V per cell (13.5V to 13.8V for a 12V battery).
A voltage that’s too high can lead to undesired side reactions like the formation of gas (“gassing voltage”) towards the end of the charging process of lead-acid batteries.
Multi-stage chargers are more efficient because they can adjust the charging voltage (and charging current) throughout the charging process.
Charging Current
The charging current also needs to be considered. The correct charging current is calculated according to the battery’s capacity.
Charging Current (A) = Battery Capacity (Ah) x Charging C-rate
Usually, manufacturers recommend charging lead-acid batteries at a rate between 0.1C to 0.25C, 10% to 25% of the battery’s Ah rating. At this rate, performance and cycle life are maximized, and charge losses and side reactions (like gas formation in lead-acid batteries) are minimized.
For lead-acid batteries, exceeding a charging current of 0.3C (30% of the Ah rating) may heat up the battery and damage its internal structure, which results in permanent capacity loss.
For LiFePO4 batteries, the recommended charging C-rate is between 0.3C and 0.5C. Most LiFePO4 can withstand a charging C-rate of 1C pretty well. However, regularly applying 1C as a charging C-rate can decrease the battery’s service life.
Charging Profile Of Lead -Acid Batteries
Lead-acid batteries like AGM and Gel usually present the “three-stage” charging profile. As the name suggests, this profile consists of three charging stages: bulk, absorption, and float. The charging curve is shown below:
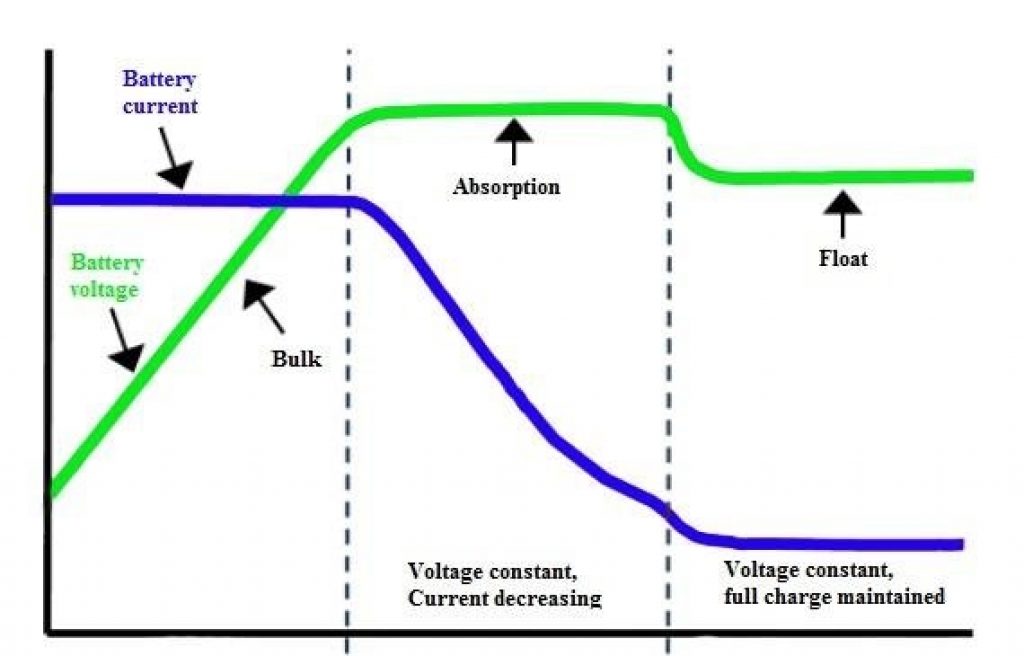
Source: Charging and Discharging Battery System Automation in Simple Wind Power Plant.
Bulk
As the curve shows, the first charging stage is Bulk. At this stage, the charger provides a constant charging current to the discharged battery while the voltage steadily increases until the absorption stage.
Absorption
During the Absorption stage, many chemicals reacted in the opposite direction (storing electrical energy as chemical energy). With this, there’s a gradual decrease in the charging current while the charging voltage is kept constant. After this stage, the battery is at about 80% SoC.
Float
In the Float stage, the voltage decreases slightly, enough to avoid overvoltage and allow the battery to charge fully. The charging current is kept at a low level so that the battery can maintain its charge (it offsets self-discharge).
Eventually, equalization and temperature compensation are also part of the charging profile of lead-acid batteries.
Therefore, the correct lead-acid battery charger should be able to provide this charging profile along with the correct charging voltage and current.
Charging Profile Of LiFePO4 Batteries
Charging a LiFePO4 battery is much simpler than charging lead-acid batteries as its charging profile does not include a float stage. Additionally, you won’t require equalization and temperature compensation.
Here’s the LiFePO4 charging curve:
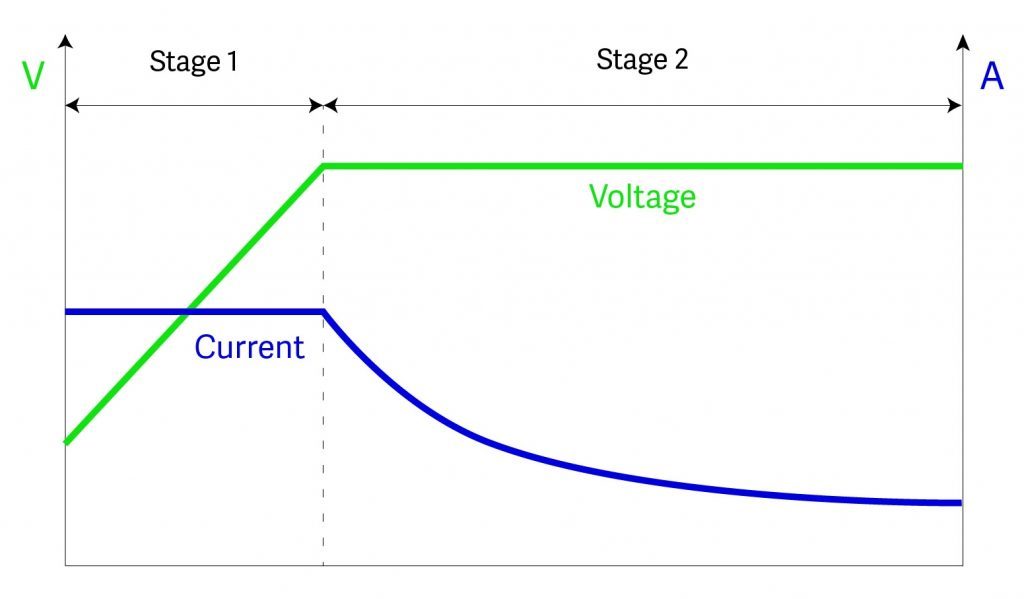
Source: Climatebiz
LiFePO4 batteries are charged according to the CC-CV charging profile. This charging profile has two stages: constant current (CC) and constant voltage (CV).
Stage 1
In the beginning, a constant charging current is applied to the battery, and the charging voltage gradually climbs until it reaches the maximum recommended charging voltage. At this point, stage 2 begins.
Stage 2
In stage 2, the charging current starts to drop while the charging voltage is kept constant. As the chemical reactions are forced in the opposite direction (i.e., opposite to the favorable discharging reactions), less current is drawn by the battery.
Once the charging current reaches about 5% of the battery’s Ah rating, the charging is cut off, and the battery is fully charged. This stage takes around 20 minutes.
Because of its lower internal resistance, LiFePO4 batteries can accept a charging current much higher than lead-acid batteries. In addition, LiFePO4 batteries are inherently more stable than lead-acid batteries, so they have a much wider voltage tolerance (i.e., charging voltage is higher).
Lithium battery chargers are usually more expensive than SLA battery chargers because they need to be able to provide high charging currents. Moreover, the technology behind these chargers is more advanced.
Smart Chargers
Modern batteries are equipped with microprocessors that “communicate” with smart chargers so that the smart charger can provide the correct charging algorithm for the battery.
Most smart chargers come with pre-programmed charging modes. When connected to the battery, the battery and charger “communicate” so the charger can provide the appropriate charging specs to charge the battery efficiently. Some chargers can even be manually programmed (via an app) with the user’s desired charging specs.
There’s a wide range of chargers: multi-stage, multi-bank, constant current, constant voltage, recovery charger, taper chargers, etc.
Charging Time
Charging time is a complicated parameter to calculate. It depends on several variables, such as:
- The battery’s age: an old battery will probably take less time to charge because of capacity loss over time.
- The charging efficiency of the battery.
- The charging profile: how long is a constant current provided? Is a float charge necessary?
- If the battery is simultaneously powering a load.
- The charging temperature (this is a huge factor when it comes to charging time).
Under normal circumstances (a healthy battery, charging at room temperature), you can estimate charging time using:
Charging Time (h) = Battery Capacity (Ah) / Charging Current (A)
Example 1: Using a 40A charger to charge a 120Ah LiFePO4 (approximately 0.3C charging C-rate) would take around 3 hours (“around” because this is only an estimation):
Charging Time (h) = 120 Ah / 40 A = 3 hours
Example 2: Using a 10A charger to charge a 100Ah AGM battery would take around 10h:
Charging Time (h) = 100 Ah / 10 A = 10 hours
You can achieve an even better estimation by adding 0.5h to the estimated charging time for LiFePO4 batteries and 1h for Lead-acid batteries. This compensates for the charging losses.
Summary: The Right Battery Charger Size
The correct battery charger should offer the appropriate charging profile for your battery, with the proper charging voltage and charging current.
Battery chargers are rated in Amps, and they also indicate what battery voltage you should use. So if you want to charge a 12V battery, use a 12V charger. For a 24V battery, use a 24V battery charger, and so on.
Now, regarding charging current, here’s what’s recommended:
The recommended charging current (thus, the battery charger size) for lead-acid batteries ranges from 0.1C to 0.25C (10% to 25% of the battery’s Ah rating). For example, if your lead-acid battery has 100Ah of capacity, you should use a charger rated for at least 10A (or anything between the 10A to 25A range).
LiFePO4 Batteries:
For LiFePO4, the charging current should be around 0.3C to 0.5C (30% to 50% of the battery’s Ah rating). A charging current of 0.5C offers a good balance between charging time and charging safety.
So for a 50Ah LiFePO4 battery, a good charger should provide a charging current between 15A and 25A. The charging current determines the charging time.
Moreover, most LiFePO4 can accept a charging current of 1C (100% of the rated battery capacity) without suffering any severe damage. However, regularly charging at a rate of 1C may eventually impact the lithium battery’s cycle life, so keeping it between 0.3C and 0.5C is the best choice.
Battery Charger Size Chart
Here are a few different battery charger size charts that can come in handy:
Charging Current
For LiFePO4 batteries:
LiFePO4 Battery Capacity | 0.3C Charging Rate (around 3.5h – 4h to charge) | 0.5C Charging Rate (approximately 2h – 2.5h to charge) | 1C Charging Rate (approximately 1h – 1.5h to charge) |
---|---|---|---|
20 Ah | 6 A | 10 A | 20 A |
50 Ah | 15 A | 25 A | 50 A |
80 Ah | 24 A | 40 A | 80 A |
100 Ah | 30 A | 50 A | 100 A |
120 Ah | 36 A | 60 A | 120 A |
150 Ah | 45 A | 75 A | 150 A |
200 Ah | 60 A | 100 A | 200 A |
250 Ah | 75 A | 125 A | 250 A |
300 Ah | 90 A | 150 A | 300 A |
For SLA (AGM And Gel) Batteries
SLA Battery Capacity | 0.1C Charging Rate (around 10 – 12h to charge) | 0.25C Charging Rate (around 8h – 10h to charge) | 0.3C Charging Rate (around 6h – 8h to charge) (0.3C is the maximum recommended) |
---|---|---|---|
20 Ah | 2 A | 5 A | 6 A |
50 Ah | 5 A | 12.5 A | 15 A |
80 Ah | 8 A | 20 A | 24 A |
100 Ah | 10 A | 25 A | 30 A |
120 Ah | 12 A | 30 A | 36 A |
150 Ah | 15 A | 37.5 A | 45 A |
200 Ah | 20 A | 50 A | 60 A |
250 Ah | 25 A | 62.5 A | 75 A |
300 Ah | 30 A | 75 A | 90 A |
Recommended Charging C-rate And Average Charging Time
Battery Chemistry | Charging Stages | Average Charging Time | Recommended Charging C-rate |
---|---|---|---|
Lead-Acid batteries (AGM and Gel) | Bulk, Absorption, and Float | Around 10 hours | Between 0.1C and 0.25C (shouldn’t exceed 0.3C) |
LiFePO4 batteries | Constant Current (CC) and Constant Voltage (CV) | Around 4 hours | Between 0.3C and 0.5C (1C is also accepted but shouldn’t be applied regularly) |
Charging Voltage
System Voltage | Charging Voltage Range (at 25ºC or 77ºF) |
---|---|
LiFePO4 12V | 14V – 14.6V |
LiFePO4 24V | 28V – 28.6V |
AGM 12V | 14.6V – 14.8V (absorption) 13.4V to 13.8V (float) |
AGM 24V | 28.4V -29.2V (absorption) 27.0V – 27.6V (float) |
Gel 12V | 14.1V to 14.4V (absorption) 13.1V to 13.4V (float) |
Gel 24V | 27.6V – 28.3V (absorption) 26.4V – 27V (float) |
Can A Battery Charger Be Too Big?
In terms of voltage, yes. For instance, you shouldn’t use a 24V charger to charge a 12V battery.
In terms of current, not quite.
If your charger is working correctly, it should be able to “communicate” with your battery to know what charging current to provide.
What’s more, the charger’s amp rating expresses the maximum current the charger can provide; it doesn’t mean that the charger can only provide this amount of current.
After all, as you saw previously, the current varies (decreases) throughout the charging process. This indicates that the charger can deliver a current lower than what it’s rated for.
Example
Suppose you have a 12V charger rated at 50A, and you want to charge a 12V 100Ah AGM battery. Given the recommended charging current range for AGM of 0.1C to 0.25C, the appropriate charging current for this battery should be between 10A and 25A.
In this case, the 50A charger would only deliver a charging current between 10A and 25A to the battery because that’s what the charging microprocessor contained in the battery would “communicate” to the charger.
To summarize, a charger can’t be “too big.” The battery will draw the current it needs, and the charger will provide the current drawn by the battery. Using a “large” charger shouldn’t damage the battery or the charger. It does impact your wallet, though.
As the amperage rating of charges increase, so does their prices. Therefore, there’s no point “investing” in a large charger if you’re never going to use its maximum current. That would be a waste of money.
Instead, choose a battery charger with an amperage rating compatible with your battery’s recommended charging current range.
Battery Management System (BMS)
In addition, even if the charger tried to deliver a charging current higher than what the battery requires, the battery’s BMS wouldn’t allow for this current to reach the battery. BMSs are programmed with a cut-off voltage and current, and the BMS cuts off anything outside the acceptable range.
Final Thoughts
Using the correct battery charger size is vital to maintaining your battery’s health.
With the right battery charger size, your battery can efficiently charge, providing high performance and maximized cycle life.
Conversely, using the wrong battery charger size can overcharge (or undercharge) your battery and, over time, cause a significant negative impact on its cycle life and overall performance.
In this article, we explained that the charging voltage depends on your battery’s voltage and the charging current depends on its capacity. Moreover, each battery chemistry has an appropriate charging profile (or charging algorithm).
This charging profile determines how the charging specs vary throughout the charging process.
Additionally, charging time is closely related to the charging current, although it’s also associated with many other variables, such as charging temperature.
At the end of the day, when choosing a battery charger, consider the chemistry of your battery, its voltage, and its capacity.